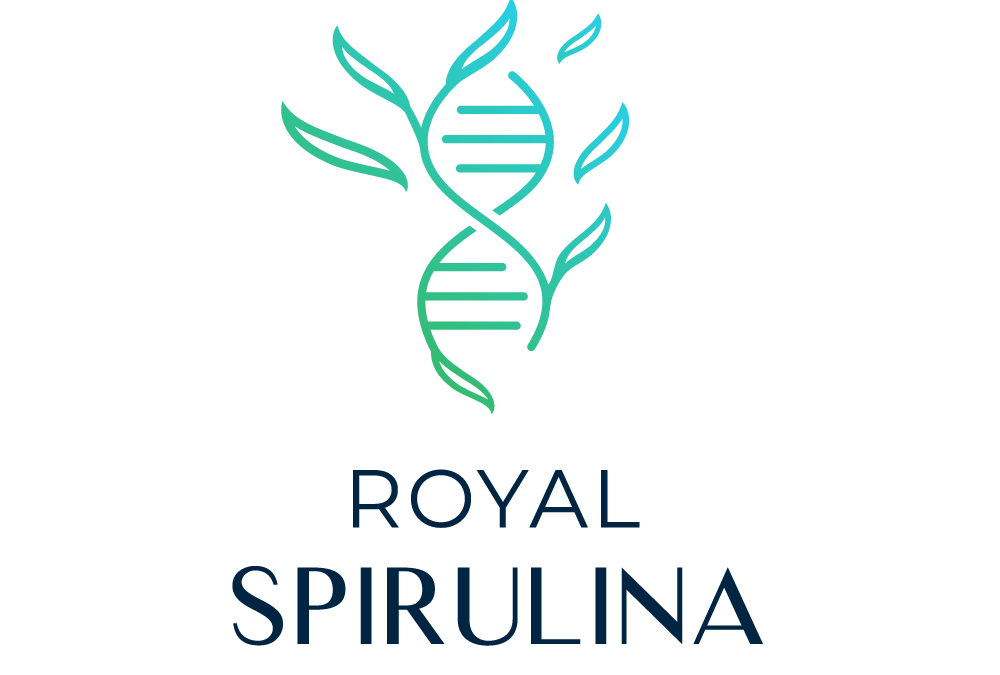
Spirulina as a Source of Prebiotics
Date: 20 August 2021- Revision No 01 CONFIDENTIAL & PROPRIETARY INFORMATION
Spirulina as a Source of Prebiotics
Objective – This project aims to evaluate the prebiotic properties of spirulina and the nutraceutical products made from it.
Introduction
Prebiotics are a group of nutrients that are metabolized and uptaken by beneficial microbes of the human gastrointestinal microbiome, promoting their growth and activity. The metabolites produced by these microbes are released into the bloodstream, affecting not only the digestive system but also distant organs around the body. Prebiotics are also a viable candidate for replacing antibiotics added to livestock feed, ensuring the health of livestock microbiota without the risk of generating antibiotic resistance in pathogenic bacteria. Scientists are attempting to produce prebiotics on an industrial scale, as they have production and storage advantages compared to probiotics (i.e. beneficial gut microbiota ingested as dietary supplements).1
The biomass of spirulina (Limnospira fusiformis), a cyanobacterium cultivated for its nutritional value, is a confirmed source of prebiotics. Nutrients in spirulina which promote the growth of beneficial gut microbes include polysaccharides, polyphenols, and polyunsaturated fatty acids.2 3 4 This investigation finds that spirulina is capable of improving and maintaining the health of the gut microbiome of various animals under modulated doses; however, further experimentation is needed to confirm the prebiotic properties of spirulina in humans. Moreover, the nutrient profile of spirulina is refined when fermented by various mixtures of probiotic species, revealing the potential for spirulina as an ingredient in synbiotic supplements (i.e. dietary supplements that synergistically combine probiotics and the prebiotics on which they feed).
Discussion
The Prebiotic Content of the Spirulina Phytocomplex
The spirulina phytocomplex contains various nutrients which stimulate the growth of gut microbes. Oligosaccharides and polysaccharides are complex carbohydrates, large molecules comprised of simple sugar monomers (i.e. “building blocks”). In the case of the spirulina phytocomplex, these monomers include glucose, fructose, glycerol, mannitol, sorbitol, rhamnose, mannose, xylose, galactose, arabinose, glucosamine, and rhamnosamine.5 6 7 Once ingested, the complex carbohydrates present in spirulina preferentially stimulate the growth of lactic acid bacteria, which are highly beneficial members of the gut microbiome, as will be discussed later in this investigation.6
Polyphenols are molecules formed from one or more aromatic rings with one or more hydroxyl (-OH) groups. Polyphenols present in the spirulina phytocomplex predominantly consist of gallic, chlorogenic, cinnamic, p-OH-benzoic, quimic, caffeic, vanillic, and ferulic acids.8 9 In addition to increasing the abundance of lactic acid bacteria, these polyphenols also increase immune tolerance to them by reducing serum concentrations of proinflammatory cytokines (i.e. intercellular messenger molecules which recruit proinflammatory immune cells).10
The spirulina phytocomplex also contains a diversity of polyunsaturated fatty acids, especially linoleic acid, γ-linolenic acid, and arachidonic acid.11 These fatty acids promote the growth of lactic acid bacteria, but only at low doses; high doses, while not lethal to gut bacteria, can hinder their normal cycle of growth and division. The polyunsaturated fatty acids in spirulina can also modify adhesion sites for gut microbes by changing the fatty acid composition of the cell membranes in gut epithelial cells (i.e. cells of the gut wall). These modifications depend on the type of polyunsaturated fatty acid: in fish, omega-3 fatty acids such as γ-linolenic acid encourage the establishment of lactic acid bacteria, while omega-6 fatty acids such as linoleic acid and arachidonic acid discourage the establishment of bacteria on the gut epithelium.12
Observed Prebiotic Properties of the Spirulina Phytocomplex
Experimental research has confirmed that the spirulina phytocomplex, when introduced whole into the gut of various animals (e.g. honey bees, quail, chickens, mice), increases the residential population of lactic acid bacteria.13 14 15 16 These bacteria secrete acidic byproducts which decrease gut pH and downregulate the gut population of acid-sensitive pathogenic bacteria (Proteus vulgaris, E. coli, etc.), a phenomenon known as the butyric effect.1 13 17 18 Lactic acid bacteria also increase the growth of other gut microbes through syntropic (nutrient-sharing) interactions.13 Spirulina also contains nutrients that inhibit the growth and spread of pathogenic bacteria (phycobiliproteins, C-phycocyanin) and viruses (calcium spirulan, cyanovirin-N), further contributing to eubiosis in the gut microbiota.19 Moreover, the gut microbiota metabolize seaweed biomass into bioactive derivatives which enter the brain via the bloodstream and modulate multiple neuronal functions. Clinical research trials are ongoing to verify whether spirulina in particular has neuroprotective and cognitive-enhancing functions.17
A recent study of the prebiotic properties of the spirulina phytocomplex in mice indicates that the abovementioned effects of spirulina consumption on the gut microbiota depend heavily on the dosage. According to this study, mice which consumed a low dose of spirulina (1.5 g/kg) showed a period of significantly decreased α-diversity (correlated with the number of microbe species) of the gut microbiome by day 14 of the treatment regimen, only to increase back to its initial α-diversity by day
21. Mice fed a high dose of spirulina (3.0 g/kg) only exhibited a steady increase in α– diversity over the 25-day regimen. Moreover, the low-dose group saw an increase in the ratio of Firmicutes to Bacteroidetes populations, indicating a state of dysbiosis in the gut microbiome which has been correlated with such diseases as obesity, irritable bowel disease, and colorectal cancer. These findings indicate that it is necessary to rationally adjust the ingested dosage of spirulina, depending on the therapeutic application. This dosing must be done for the overall homeostasis and holistic interaction of the gut microbiota rather than the modulation of select bacterial species.16
Table 1 below summarizes the findings of the present investigation into the prebiotic properties of spirulina.
Table 1
Prebiotic Benefits and Potential Applications of Spirulina
Condition |
Potential Applications |
Benefits |
Limitations |
Other Considerations |
Unfermented |
Prebiotic |
Increased population |
Prebiotic efficiency |
Whether a |
|
|
of lactic acid |
dependent on |
recommended serving |
|
|
bacteria upon |
dosage, with high |
size is necessary for |
|
|
consumption |
dosages favored |
spirulina products |
|
|
|
(e.g. ≥3.0 g/kg in |
based on the dosage |
|
|
|
mice) |
limitation of spirulina |
|
|
Increased α-diversity |
Lacking |
Whether prebiotic |
|
|
of gut microbiota |
comprehensive |
properties of spirulina |
|
|
|
verification via |
change upon |
|
|
|
human studies |
processing into |
|
|
|
|
nutraceutical products |
|
Cryoprotectant |
Enhances probiotic |
N/A |
N/A |
|
of probiotics |
viability during |
|
|
|
|
processing, |
|
|
|
|
comparable to skim |
|
|
|
|
milk |
|
|
|
|
Hypoallergenic |
|
|
Fermented |
Prebiotic |
Increased prebiotic |
Lacking |
How fermentation of |
|
|
nutrients (flavonoids |
verification via |
spirulina impacts its |
|
|
and polyphenols) |
animal and human |
dosage limitation, and |
|
|
relative to |
in vivo studies |
hence, the |
|
|
unfermented |
|
recommended serving |
|
|
spirulina |
|
size of its |
|
|
Increased population |
|
nutraceutical products |
|
|
of lactic acid |
|
|
|
|
bacteria upon |
|
|
|
|
consumption (?) |
|
|
|
|
Increased α-diversity |
|
|
|
|
of gut microbiota (?) |
|
|
|
|
Decreased levels of |
|
|
|
|
offensive-tasting |
|
|
|
|
chemicals in |
|
|
|
|
spirulina biomass |
|
|
|
Cryoprotectant |
Enhances probiotic |
Lacking |
How fermentation of |
|
of probiotics |
viability during |
verification via |
spirulina impacts its |
|
|
processing, |
animal and human |
hypoallergenicity and |
|
|
comparable to skim |
studies |
its performance as |
|
|
milk (?) |
|
probiotic |
Hypoallergenic (?)
Synbiotic N/A Lacking verification via animal and human studies cryoprotectant
Whether additional procedures, ingredients, and equipment are required to maximize viability of fermented spirulina as a synbiotic
The expiry date of a synbiotic made from fermented spirulina and human gut microbiota
Conclusion & Future Research
Although studies have revealed the overall beneficial effect of spirulina on the gut microbiota of various animals, human studies are lacking as yet. The prebiotic properties of spirulina should be deeply analyzed by human in vivo studies to confirm the benefits and risks exhibited in other animal species.16 Knowing the effect of the microbiota on the bioavailability and biotransformation of spirulina in the gut, could be crucial for understanding its effect on overall health.1617 Additionally, further experimentation on the selectivity of spirulina components to particular bacterial species may provide insights into the development of novel, nutraceutical-based, targeted drug delivery systems. Moreover, evaluating the therapeutic outcomes of spirulina treatment in human trials must utilize metabolomics, metagenomics, and transcriptomics; it must also account for such factors as dose regimen (short vs. long duration), sex (male vs. female), and the biogeography of gut microbiota (distal vs. proximal colon).16
The finding that gut microbiota metabolize seaweed biomass (incl. cyanobacteria) into byproducts which are used by the body is also worthy of further clinical research. Recent studies indicate that spirulina is nutritionally enriched upon fermentation by bacteria normally present in the human gastrointestinal tract, including Bacillus species, lactic acid bacteria, and mixtures of the former two groups. Fermented spirulina can display the following nutrient enhancements compared to its unfermented counterpart, depending on the bacterial species used in the fermentation process: significantly increased amino acid content, and ratio of essential amino acids to total amino acids; reduced levels of offensive-flavored components; increased flavonoids and polyphenols; and significantly enhanced free radical scavenging and total antioxidant ability.20
In light of these findings, further clinical research must be done to confirm the efficacy of fermented spirulina as a prebiotic component in synbiotic supplements. Such confirmation would be significant, as prebiotic fermentation would be a viable means to: a) amplify the prebiotic properties of spirulina, potentially affecting the gut microbiome more rapidly than unfermented spirulina; b) increase the nutritional benefits of spirulina consumption; and c) increase the palatability of spirulina and its products by reducing levels of offensive-tasting molecules. Additionally, the fermented spirulina could potentially enhance the viability of the introduced probiotics during processing (likely due to its high amino acid content), potentially replacing allergenic skim milk as a cryoprotectant for probiotics.21
1 Davani-Davari, Dorna, et al. “Prebiotics: Definition, Types, Sources, Mechanisms, and Clinical Applications.” Foods (Basel, Switzerland), MDPI, 9 Mar. 2019, www.ncbi.nlm.nih.gov/pmc/articles/PMC6463098/.
2 Hicks, McKenna, et al. “De-Novo Assembly of Limnospira fusiformis Using Ultra-Long Reads.” Frontiers in Microbiology, Frontiers, 16 Apr. 2001, www.frontiersin.org/articles/10.3389/fmicb.2021.657995/full.
3 Hu, Jinlu, et al. “Dose Effects of Orally Administered Spirulina Suspension on Colonic Microbiota in Healthy Mice.” Frontiers in Cellular and Infection Microbiology, Frontiers Media S.A., 5 July 2019, www.ncbi.nlm.nih.gov/pmc/articles/PMC6624478/.
4 Wang, Bingyue, et al. “Extraction of Polysaccharide from Spirulina and Evaluation of Its Activities.” Evidence-Based Complementary and Alternative Medicine, Hindawi, 11 Apr. 2018, www.hindawi.com/journals/ecam/2018/3425615/.
5 Gupta, Sneh, et al. “Prebiotic Efficiency of Blue Green Algae on Probiotics Microorganisms.” Journal of Microbiology & Experimentation, MedCrave Publishing, 6 Apr. 2017, medcraveonline.com/JMEN/prebiotic-efficiency-of-blue-green-algae-on-probiotics- microorganisms.html.
6 Wang, Bingyue, et al. “Extraction of Polysaccharide from Spirulina and Evaluation of Its Activities.” Evidence-Based Complementary and Alternative Medicine, Hindawi, 11 Apr. 2018, www.hindawi.com/journals/ecam/2018/3425615/.
7 Falquet, Jacques. “The Nutritional Aspects of Spirulina.” My Complete Health, Ian Miller, 8 Jan.
2020, www.mycompletehealthnd.com/blog/the-nutritional-aspects-of-spirulina.
8 Machu, Ludmila, et al. “Phenolic Content and Antioxidant Capacity in Algal Food Products.” Molecules (Basel, Switzerland), MDPI, 12 Jan. 2015, www.ncbi.nlm.nih.gov/pmc/articles/PMC6272319/.
9 El-Baky, HHA, et al. “Production of Phenolic Compounds from Spirulina maxima Microalgae and Its Protective Effects.” African Journal of Biotechnology, 2009, www.ajol.info/index.php/ajb/article/view/68794.
10 Alves-Santos, Aline Medeiros, et al. “Prebiotic Effect of Dietary Polyphenols: A Systematic Review.” Journal of Functional Foods, Elsevier, 21 Sept. 2020, www.sciencedirect.com/science/article/pii/S1756464620303935.
11 Diraman, Harun, et al. “Fatty Acid Profile of Spirulina Platensis Used as a Food Supplement.” EVols at University of Hawaii at Manoa: Home, Israeli Journal of Aquaculture – BAMIGDEH, 1 Jan. 1970, https://evols.library.manoa.hawaii.edu/handle/10524/19280.
12 Kankaanpää, Pasi E., et al. “Influence of Polyunsaturated Fatty Acids on Probiotic Growth and Adhesion.” OUP Academic, Oxford University Press, 1 Jan. 2001, academic.oup.com/femsle/article/194/2/149/489490.
13 Ricigliano, Vincent A., and Michael Simone-Finstrom. “Nutritional and Prebiotic Efficacy of the Microalga Arthrospira platensis (Spirulina) in Honey Bees.” Apidologie, Springer Paris, 8 May 2020, link.springer.com/article/10.1007/s13592-020-00770-5.
14 Hajati, H, et al. “Arthrospira (Spirulina) platensis Can Be Considered as a Probiotic Alternative to Reduce Heat Stress in Laying Japanese Quails.” Brazilian Journal of Poultry Science, Fundação APINCO De Ciência e Tecnologia Avícolas, 5 June 2020, www.scielo.br/j/rbca/a/4cwRyWmNWkJRWmJgkLSCgQb/?lang=en.
15 Jamil, A. B. M. Rawshon, et al. “Prebiotic Competence of Spirulina on the Production Performance of Broiler Chickens.” Journal of Advanced Veterinary and Animal Research, Bangladesh Journals Online, 2015, www.banglajol.info/index.php/JAVAR/article/view/24862.
16 Hu, Jinlu, et al. “Dose Effects of Orally Administered Spirulina Suspension on Colonic Microbiota in Healthy Mice.” Frontiers in Cellular and Infection Microbiology, Frontiers Media S.A., 5 July 2019, www.ncbi.nlm.nih.gov/pmc/articles/PMC6624478/.
17 Sorrenti, Vincenzo, et al. “Spirulina Microalgae and Brain Health: A Scoping Review of Experimental and Clinical Evidence.” MDPI, Multidisciplinary Digital Publishing Institute, 22 May 2021, www.mdpi.com/1660-3397/19/6/293/htm.
18 Byrne, Jane. “Establishing the Pig’s Gut Microbiota as Early as Possible Is Key, Says a Swine Specialist.” Feednavigator.com, William Reed Business Media Ltd., 4 July 2018, www.feednavigator.com/Article/2018/07/04/Establishing-the-pig-s-gut-microbiota-as-early-as– possible-is-key-says-a-swine-specialist.
19 Nuhu, Abdulmumin A. “Spirulina (Arthrospira): An Important Source of Nutritional and Medicinal Compounds.” Journal of Marine Biology, Hindawi, 8 May 2013, www.hindawi.com/journals/jmb/2013/325636/.
20 Yu, J., et al. “Effects of Different Probiotic Combinations on the Components and Bioactivity of Spirulina.” Journal of Basic Microbiology, U.S. National Library of Medicine, 18 Mar. 2020, pubmed.ncbi.nlm.nih.gov/32187728
21 Kordowska-Wiater, M., et al. “Spirulina Enhances the Viability of Lactobacillus rhamnosus E/N After Freeze-Drying in a Protective Medium of Sucrose and Lactulose.” Letters in Applied Microbiology, U.S. National Library of Medicine, 23 May 2011, pubmed.ncbi.nlm.nih.gov/21535045/.
Recent Comments